The Latin phrase “mens sana in corpore sano,” meaning “a healthy mind in a healthy body,” was first coined by the Roman poet Juvenal in his tenth satire. This timeless idea underscores the importance of balancing mental and physical health for a fulfilling life. In stark present contrast, metabolic syndrome is becoming a widespread public health problem.
Metabolic syndrome is often caused by an unbalanced diet combined with too little physical inactivity. It is a collection of metabolic disorders that can lead to neurocognitive dysfunction by triggering inflammatory mediators, disrupting brain metabolism and impairing cognitive function. In addition to its direct effects on brain tissue, metabolic syndrome can also cause indirect damage.
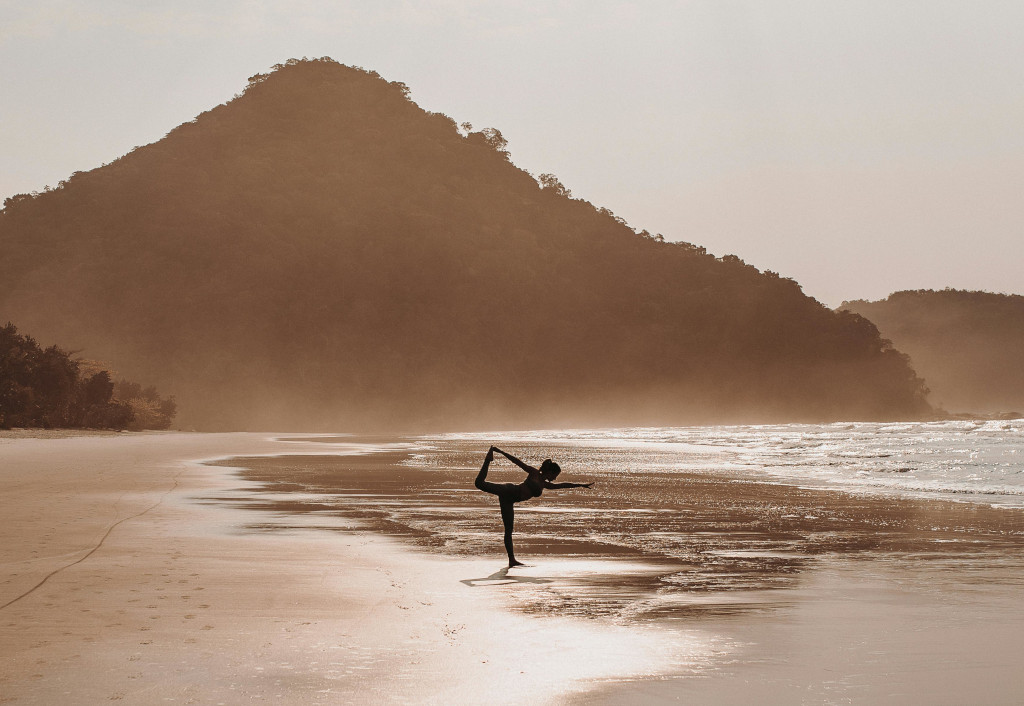
For example, evidence shows that a sedentary lifestyle reduces oxygenation and blood flow, leading to long-term neural damage. To address these issues, both preventive and therapeutic cognitive-behavioural interventions are designed to facilitate healthy lifestyle changes and improve brain function, thereby enhancing overall quality of life.
How Does The Brain Change In Metabolic Syndrome?
Metabolic Syndrome (MetS) constitutes a significant contributor to illness and mortality. It hence represents a significant threat to public health. This condition is closely linked to an increased risk of non-communicable diseases (NCDs) such as cardiovascular disease and type 2 diabetes. Beyond its serious health consequences, MetS diminishes the quality of life and places a growing economic burden on healthcare systems (1).
An emerging concern is the association between MetS and neurocognitive dysfunctions, which pose a significant challenge (2, 3). In this context, certain molecules mediating communication between cells appear to be key players in pathways that trigger metabolic inflammation and microvascular disorders. This could lead to damage brain white matter and a decline in cognitive function (4).
Furthermore, insulin resistance – a critical component of MetS – can lead to variations in blood glucose levels and elevated serum insulin. The impairment of insulin signalling in the brain, coupled with oxidative stress, can lead to biochemical modification, specifically glycation, of brain tissue and from there, subsequent brain inflammation (5). In addition, high glucose levels in the brain have been linked to cognitive impairments, including dementia. Moreover, well-known contributors to MetS like obesity and hypertension are considered risk factors both for dementia and severe brain degeneration (6).
Controlling MetS can slow the degeneration of both white and grey matter in the brain (7, 8). In addition to its role in diabetes and cerebrovascular disorders, MetS has also been identified as a risk factor for the progression of Alzheimer’s disease (9). MetS can accelerate the onset of cerebral small vessel dysfunctions causing structural and functional changes in blood vessels, leading to mild bleeding, white matter damage and brain atrophy (10), the latter most often in deep white matter areas, such as the right frontal lobe (5).
MetS can also have an indirect impact on the brain. A sedentary lifestyle is a key risk factor, as inactivity leads to reduced oxygenation and circulation, which can cause long-term neural damage. A change in lifestyle and promotion of physical activity can lead to a notable enhancement in the oxygenation of the brain, thus improving its function overall.
Shedding Light On Cognitive Impairment
Some studies have indicated a reduction in both attention and neuromuscular connections in elderly patients with MetS when performing cognitive tasks, particularly those associated with the parietal and occipital lobes (11, 12). Based on the results of numerous studies investigating the impact of MetS on brain structure and cognitive ability (7), it can be concluded that obesity, hypertension and hyperglycaemia have the greatest impact on reducing cognitive performance and ability (5, 13).
A decline in cognitive function associated with MetS is characterised by the following symptoms: reduced decision-making ability, reduced memory function and a decline in the reward system (14). It should be noted that MetS is not the only cause of impaired brain metabolism and the resulting cognitive and structural changes. At present, there is a growing focus on metabolic disorders among those studying metabolic diseases. Furthermore, the high prevalence of MetS and diabetes mellitus, coupled with the slowing of metabolism in all organs – including the brain – has led to an increased focus on MetS.
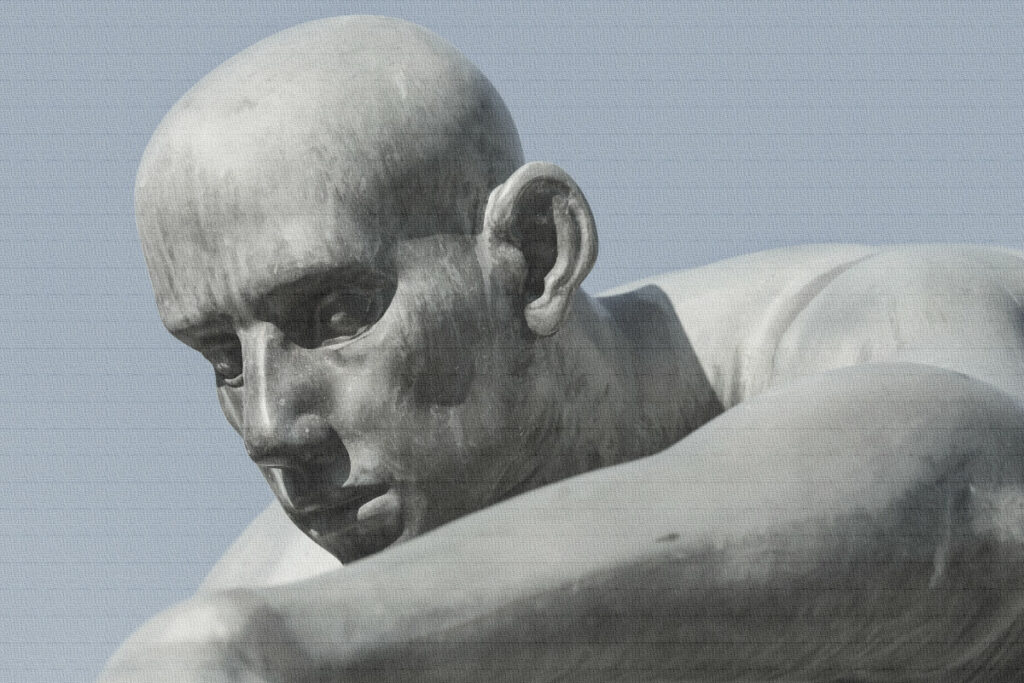
Brain metabolism is known to play a major role in causing certain neurological diseases, such as Alzheimer’s (15-17). This is due to hypometabolism – a condition where the brain uses less energy than normal – which promotes the buildup of a protein called “amyloid-beta” (Aβ) in the brain (18). Normally, this protein is cleared away but when it accumulates it can form clumps or plaques, that interfere with how brain cells communicate and can cause brain cell damage. Additionally, proton magnetic resonance spectroscopy has revealed alterations in metabolite concentration. A significant correlation has been identified between cognitive decline and neurometabolic disorders in various areas of the brain, including multiple sclerosis (19), brain tumours (20, 21), epilepsy (22, 23), Alzheimer’s disease (24-26) and dementia (27).
It has been established that the majority of metabolic diseases affecting the brain result in structural changes to neurons, which can lead to a decline in cognitive abilities. Some studies have demonstrated that patients with obesity exhibit a notable reduction in grey matter volume in various regions of the brain, including the cerebellum and thalamus, while also displaying an increase in grey matter volume in the pallidum and hippocampus (28-30).
Neurocognitive Approaches
Scientists are investigating the potential of neurocognitive interventions – which are techniques or treatments that target the brain’s functioning – to facilitate healthy behaviours or reduce harmful ones in patients with MetS. A study by researcher Garcia-Silva and colleagues evaluated the efficacy of 48 cognitive behavioural interventions in volunteers with MetS (31). These included techniques for effective refusal, anger control and appetite control. Following a period of three to six months, biochemical indices and Body Mass Index (BMI) were reassessed, revealing significant changes in these indices. The primary outcome resulting from the interventions was the ability of individuals to adhere to a diet for metabolic syndrome (31).
It has been proven that integrating cognitive interventions with other strategies, such as promoting physical activity, can result in sustained changes in children’s behaviour, habits and lifestyle. Furthermore, researchers noted a significant increase in grey matter volume in the cerebellum and total brain volume in patients with obesity when following such a holistic intervention, in comparison to healthy children (33).
The mechanisms underlying the effects of cognitive interventions have also been evaluated. A study by Shigaeff and collaborators on a cohort of adult volunteers with MetS revealed a significant increase in grey matter volume and total brain mass via a modification of food choices and dietary behaviours (11). In addition, a recent meta-analysis has also demonstrated that cognitive therapy significantly reduced binge-eating episodes and abstinence from binge-eating (34). In any case, there are encouraging reasons to see promise in neurocognitive interventions promoting structural changes in the brain—an opportunity that should be further tapped into.
Best Ways Of Designing Effective Interventions
To design effective interventions it is important to look at the behaviours associated with metabolic syndrome from different perspectives. Firstly, it is essential to address the behaviours associated with MetS, including high-risk ones such as unhealthy nutritional habits and sedentary lifestyle. The majority of interventions in this field are cognitive behavioural, to instil positive beliefs, and encourage healthy behaviours and lifestyles.
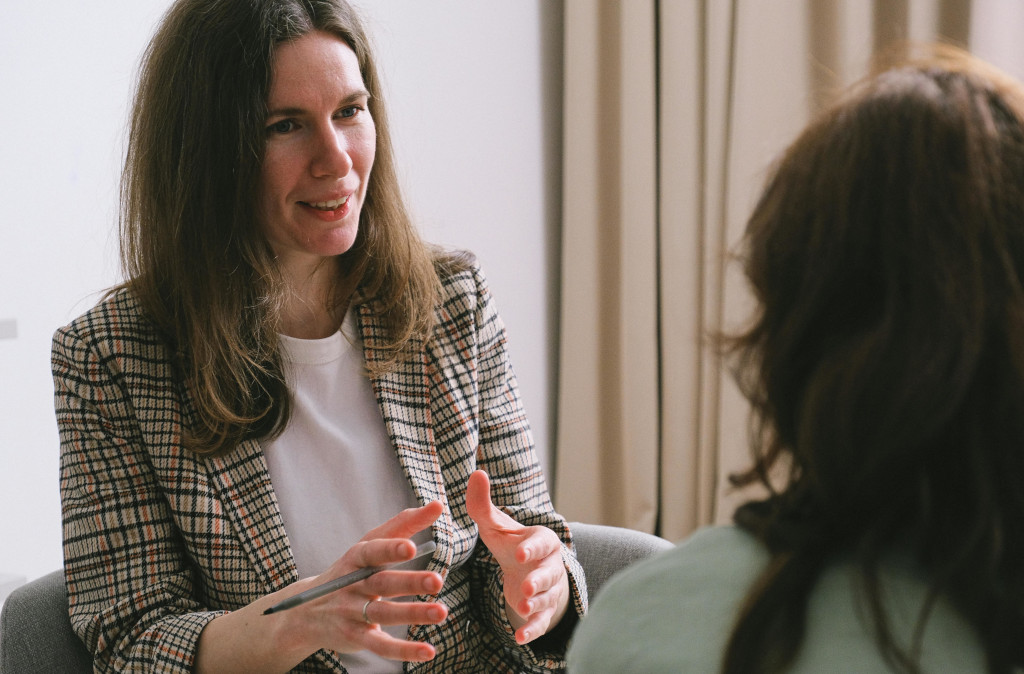
Secondly, behavioural changes resulting from cognitive impairment are linked to structural anomalies in the brain caused by MetS (1,7). For instance, the impact of MetS on memory has been demonstrated in multiple studies, with this cognitive impairment potentially resulting in significant behavioural issues for patients (12-14).
It is recommended that both preventive and therapeutic interventions are added to clinical guidelines for MetS management. This will help prevent the behavioural disorders caused by cognitive impairments in patients. The identification of structural brain correlates of health-related behaviours provides a foundation for the development of more effective behavioural interventions. This is achieved by mapping the corresponding brain regions and implementing brain-targeted behavioural interventions (31-33).
In light of all this, it is on the one hand important to consider the various disorders that may be present when developing cognitive-behavioural interventions for MetS. On the other, large-scale studies should be addressed to gain a full understanding of the structural changes in the brain of people with MetS, the associated behaviours and their management.
Cognitomics: the Link of Cognitive Function and Disease
The majority of neurocognitive interventions for metabolic syndrome have historically sought to modify lifestyles and reduce risky behaviours. However, there has been a notable lack of focus on the neural mechanisms essential for maintaining these healthy behaviours, a topic explored within social neuroscience. It is becoming increasingly clear that key brain regions, such as the superior medial frontal cortex, play a vital role in controlling behaviours like appetite. There is a growing consensus that improving the function of these areas could be crucial for achieving lasting behaviour change. To this end, effective interventions should first pinpoint and target the neural bases of the desired change.
Bibliography
- Potenza MV, Mechanick JI. The metabolic syndrome: definition, global impact, and pathophysiology. Nutr Clin Pract 2009; 24:560-77.
- Qavam SE, Anisan A, Fathi M, Pourabbasi A. Study of relationship between obesity and executive functions among high school students in Bushehr, Iran. J Diabetes Metab Disord 2015; 14.
- Pourabbasi A, Tehrani-Doost M, Qavam SE, Arzaghi SM, Larijani Association of diabetes mellitus and structural changes in the central nervous system in children and adolescents: a systematic review. Diabetes Metab Disord 2017; 16:10.
- Yates KF, Sweat V, Yau PL, Turchiano MM, Convit A. Impact of metabolic syndrome on cognition and brain: a selected review of the literature. Arterioscler Thromb Vasc Biol 2012; 32:2060-7.
- Alfaro FJ, Gavrieli A, Saade-Lemus P, Lioutas VA, Upadhyay J, Novak V. White matter microstructure and cognitive decline in metabolic syndrome: a review of diffusion tensor imaging. Metabolism 2018; 78:52-68.
- Wang H, Wen B, Cheng J, Li H. Brain structural differences between normal and obese adults and their links with lack of perseverance, negative urgency, and sensation seeking. Sci Rep 2017; 7:40595.
- Mellendijk L, Wiesmann M, Kiliaan AJ. Impact of nutrition on cerebral circulation and cognition in the metabolic syndrome. Nutrients 2015; 7:9416-39.
- Lampe L, Zhang R, Beyer F, Huhn S, Kharabian Masouleh S, Preusser S, et al. Visceral obesity relates to deep white matter hyperintensities via inflammation. Ann Neurol 2019; 85:194-203.
- Cantero MD, Villa Etchegoyen C, Perez PL, Scarinci N, Cantiello HF. Bundles of brain microtubules generate electrical oscillations. Sci Rep 2018; 8:11899.
- Yaffe K. Metabolic syndrome and cognitive disorders: is the sum greater than its parts? Alzheimer Dis Assoc Disord 2007; 21:167-71.
- Shigaeff N, Amaro E, Franco FG, Jacinto AF, Chiochetta G, Cendoroglo MS, et al. Functional magnetic resonance imaging response as an early biomarker of cognitive decline in elderly patients with metabolic syndrome. Arch Gerontol Geriatr 2017; 73:1-7.
- Shigaeff N, Jacinto A, Chiochetta G, Cendoroglo MS, Amaro E, Ikeda M, et al. Cognition and cerebral neurovascular coupling in the elderly with metabolic syndrome. Alzheimers Dement 2012; 8:P492.
- Joo SH, Yun SH, Kang DW, Hahn CT, Lim HK, Lee CU. Body mass index in mild cognitive impairment according to age, sex, cognitive intervention, and hypertension and risk of progression to Alzheimer’s disease. Front Psychiatry 2018; 9:142.
- Alarcón G, Ray S, Nagel BJ. Lower working memory performance in overweight and obese adolescents is mediated by white matter microstructure. J Int Neuropsychol Soc 2016; 22:281-92.
- Bero AW, Yan P, Roh JH, Cirrito JR, Stewart FR, Raichle ME, et al. Neuronal activity regulates the regional vulnerability to amyloid-β deposition. Nat Neurosci 2011; 14:750-6.
- Mosconi L. Glucose metabolism in normal aging and Alzheimer’s disease: methodological and physiological considerations for PET studies. Clin Transl Imaging 2013; 1:217-33.
- Bero AW, Yan P, Roh JH, Cirrito JR, Stewart FR, Raichle ME, et al. Neuronal activity regulates the regional vulnerability to amyloid-β deposition. Nat Neurosci 2011; 14:750-6.
- Chételat G, Landeau B, Salmon E, Yakushev I, Bahri MA, Mézenge F, et al. Relationships between brain metabolism decrease in normal aging and changes in structural and functional connectivity. Neuroimage 2013; 76:167-77.
- Sun J, Song H, Yang Y, Zhang K, Gao X, Li X, et al. Metabolic changes in normal appearing white matter in multiple sclerosis patients using multivoxel magnetic resonance spectroscopy imaging. Medicine (Baltimore) 2017; 96:e6534.
- Carlin D, Babourina-Brooks B, Davies NP, Wilson M, Peet AC. Variation of T2 relaxation times in pediatric brain tumors and their effect on metabolite quantification. J Magn Reson Imaging 2019; 49:195-203.
- Julià-Sapé M, Candiota AP, Arús C. Cancer metabolism in a snapshot: MRS(I). NMR Biomed 2019; 32:e4054.
- Dhamala E, Abdelkefi I, Nguyen M, Hennessy TJ, Nadeau H, Near J. Validation of in vivo MRS measures of metabolite concentrations in the human brain. NMR Biomed 2019; 32:e4058.
- Reddy SD, Younus I, Sridhar V, Reddy DS. Neuroimaging biomarkers of experimental epileptogenesis and refractory epilepsy. Int J Mol Sci 2019; 20:220.
- Kantarci K, Jicha GA. Development of 1H MRS biomarkers for tracking early predementia Alzheimer disease. Neurology 2019; 9:209-10.
- Oeltzschner G, Wijtenburg SA, Mikkelsen M, Edden RA, Barker PB, Joo JH, et al. Neurometabolites and associations with cognitive deficits in mild cognitive impairment: a magnetic resonance spectroscopy study at 7 Tesla. Neurobiol Aging 2019; 73:211-8.
- Zhu XH, Lu M, Chen W. Advanced multinuclear magnetic resonance spectroscopy (MRS) imaging approaches for studying brain metabolism, neuroenergetics, and function. In: Iaizzo PA, editor. Engineering in medicine: advances and challenges. Cambridge: Academic Press; 2019. p. 463-91.
- Crosson Bruce. Imaging in “healthy” aging and dementia: a bigger sandbox (lecture presented on March 28, 2018 at the Georgia Tech Student Center. Available from: https://repository.gatech.edu/entities/publication/531e4dbb-6a1e-4d37-824d-9d6695d370f7
- de Groot CJ, van den Akker EL, Rings EH, Delemarre-van de Waal HA, van der Grond J. Brain structure, executive function and appetitive traits in adolescent obesity. Pediatr Obes 2017; 12:e33-6.
- Moreno-López L, Soriano-Mas C, Delgado-Rico E, Rio-Valle JS, Verdejo-García A. Brain structural correlates of reward sensitivity and impulsivity in adolescents with normal and excess weight. PLoS One 2012; 7:e49185.
- Ou X, Andres A, Pivik RT, Cleves MA, Badger TM. Brain gray and white matter differences in healthy normal weight and obese children. J Magn Reson Imaging 2015; 42:1205-13.
- Garcia-Silva J, Navarrete NN, Peralta-Ramírez MI, García-Sánchez A, Ferrer-González MÁ, Caballo VE. Efficacy of cognitive behavioral therapy in adherence to the mediterranean diet in metabolic syndrome patients: a randomized controlled trial. J Nutr Educ Behav 2018; 50:896-904.
- Grilo CM, Masheb RM, Wilson GT, Gueorguieva R, White MA. Cognitive-behavioral therapy, behavioral weight loss, and sequential treatment for obese patients with binge-eating disorder: a randomized controlled trial. J Consult Clin Psychol 2011; 79:675-85.
- Augustijn MJ, D’Hondt E, Leemans A, Van Acker L, De Guchtenaere A, Lenoir M, et al. Weight loss, behavioral change, and structural neuroplasticity in children with obesity through a multidisciplinary treatment program. Hum Brain Mapp 2019; 40:137-50.
- Hilbert A, Petroff D, Herpertz S, Pietrowsky R, Tuschen-Caffier B, Vocks S, et al. Meta-analysis of the efficacy of psychological and medical treatments for binge-eating disorder. J Consult Clin Psychol 2019; 87:91-105.