Managing blood glucose levels is a crucial part of life for individuals with diabetes. While traditional methods are effective, they can be invasive and inconvenient, making full adherence to consistent monitoring a challenge. Emerging biofluid-based glucose sensing technologies offer a promising alternative. These devices analyse glucose levels in biofluids such as saliva, tears, sweat, and interstitial fluid. Since these fluids contain measurable amounts of glucose, biosensors can effectively assess blood glucose levels, albeit indirectly.
These emerging technologies eliminate the need for frequent finger pricks, representing a significant advancement in diabetes care and reflecting broader progress in digital health and medical innovation. This aligns with the growing demand for more comfortable and accessible healthcare solutions. While still evolving, these developments signal a shift towards smarter, less intrusive ways to support individuals in managing their health, with an emphasis on convenience, ease of use, and accuracy.
Diabetes mellitus is a serious and rapidly increasing global health issue (1, 2). It is characterised by persistently elevated blood glucose levels resulting from a failure in insulin production, reduced insulin sensitivity and function, or both (3). This condition places a significant burden on society and healthcare systems while profoundly impacting the quality of life and life expectancy of those affected. Diabetes can lead to various complications, including retinopathy, renal failure, atherosclerosis, and stroke (4).
Meals and activities significantly influence blood glucose levels, making continuous glucose monitoring an invaluable tool for patients. Such monitoring helps individuals better understand how their glucose levels respond to various factors. By tracking the trends and fluctuations, patients can achieve more comprehensive and effective diabetes management.
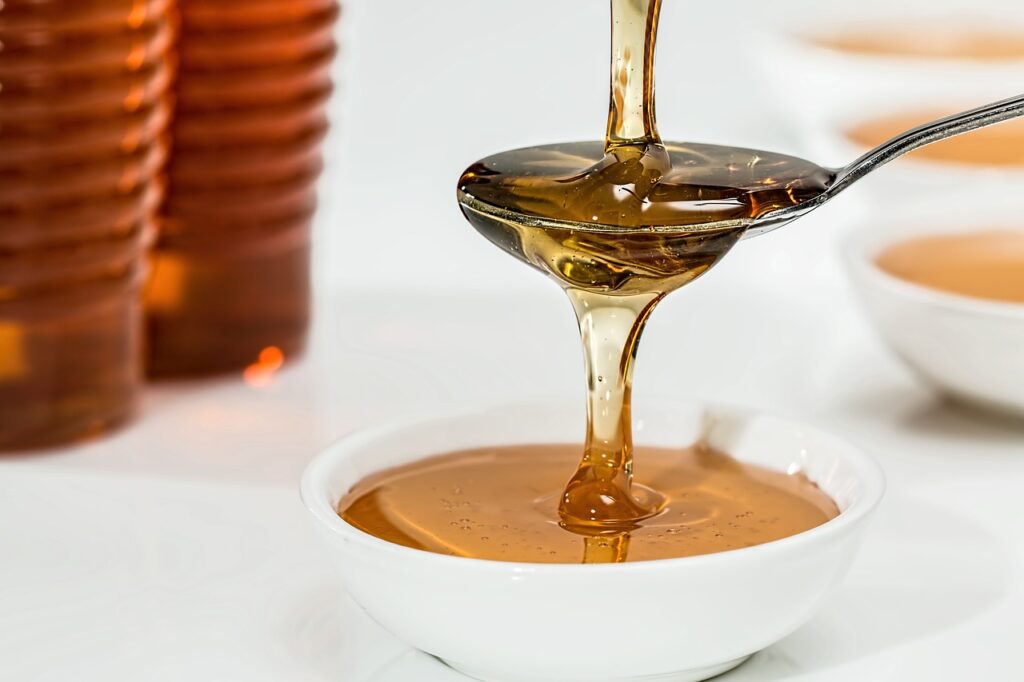
Conventional blood glucose detection techniques remain widely regarded as the gold standard, as highlighted by a recent patent analysis conducted by FRESCI. Self-monitored glucose refers to measuring glucose concentration at a specific point in time, typically with a home glucose monitor (5).
Home blood glucose monitors commonly utilise glucose oxidase biosensors, drawing blood from the fingertip (after pricking the finger) via disposable paper strips and determining glucose concentration through the chemical reaction current of the strip. Prominent commercial brands of blood glucose meters include Roche, Sano, Omron, Johnson & Johnson, Bayer, Abbott, Echeng, and Ecco (6). However, such devices are acknowledged to be invasive, as they can cause discomfort to patients.
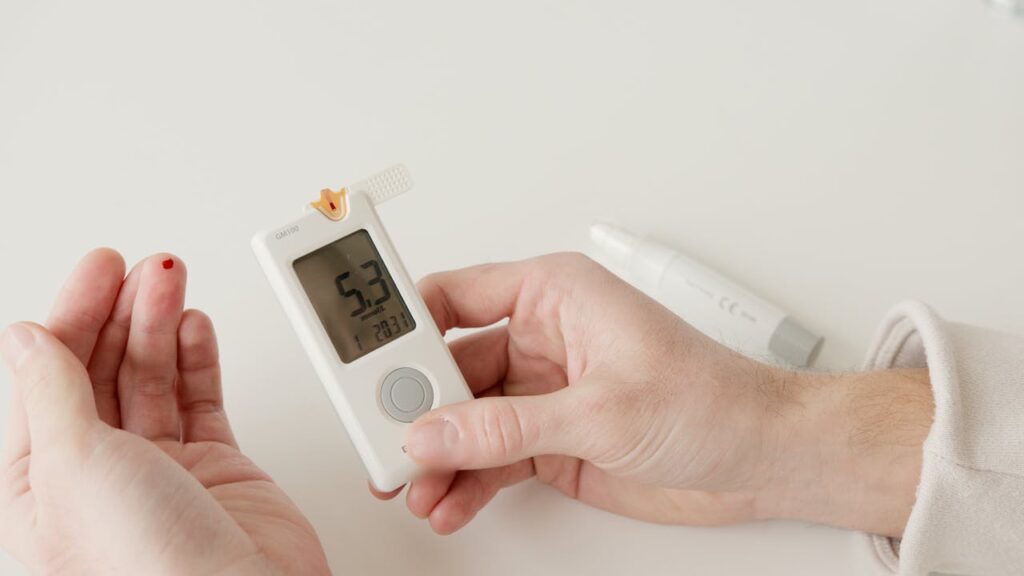
This has led to a growing focus on the development and implementation of non-invasive techniques in recent years (6–10). Among these, the two most popular approaches are based on spectral and electrochemical principles.
Glucose in aqueous solution, as happens in biological fluids, possesses a high refractive index, allowing it to bend light more effectively than many other biological substances—a property that facilitates its detection. Additionally, the specific absorption band of glucose in the near-infrared spectrum can be quantified using spectroscopic techniques.
In contrast, electrochemical methods measure the electrical signals generated by the oxidation or reduction of glucose molecules (like in blood-based glucose oxidase sensors). These methods are characterised by their high sensitivity and specificity, making them particularly well-suited for non-invasive glucose monitoring.
Smart Tech For Glucose Monitoring: The Non-Invasive Breakthrough
The key to successful non-invasive glucose monitoring lies in exploring biofluids such as saliva, tears, sweat, and interstitial fluid, which strongly correlate with blood glucose levels. These biofluids are easier to collect than blood and do not cause discomfort during the procedure. Additionally, they can be obtained at any time, enabling the continuity of glucose monitoring.
Among these biofluids, saliva stands out as an accessible option for non-invasive monitoring. A salivary glucose sensor is typically designed as a mouthguard made from biocompatible and non-toxic materials (11–13). Saliva, which is easier to collect than other biofluids, contains a range of biomarkers, including glucose, lactic acid, phosphate, enzymes (e.g., amylase), hormones (e.g., cortisol and steroids), and antibodies. However, environmental factors like temperature and humidity can promote bacterial growth in the mouth, potentially compromising sensor sensitivity. To address this, researchers are developing novel biomaterials with enhanced antifouling properties and sensitivity (14, 15).
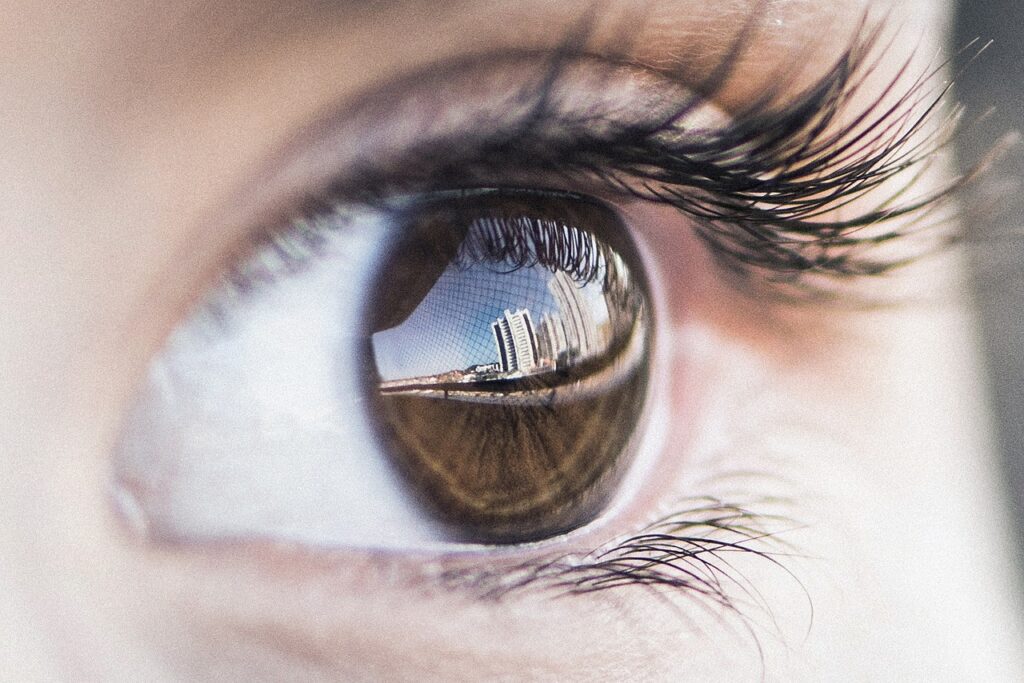
Tears are also a fluid rich in biomarkers, containing significant quantities of salt, protein, enzymes, and glucose. The main advantage of tear-based non-invasive glucose sensors is that tears contain fewer interfering contaminants, and their glucose levels correlate positively with blood glucose (16). Currently, many research teams are focusing on contact lens glucose sensors for user portability and sustainability in glucose monitoring (17–19). In addition to non-invasive continuous glucose detection, these systems can also transmit real-time data to the user’s mobile device via wireless transmission (20).
Glucose sensing based on sweat has also been a major focus of research in recent years. Sweat, secreted by sweat glands, contains metabolites, electrolytes, trace elements, and biological macromolecules. The presence of blood-related biomarkers in sweat makes it a promising medium for non-invasive monitoring (21). Their implementation using patches or band-type sensors (e.g., wristbands or abdominal bands) offers significant advantages, including easy sampling and integration into wearable technology. These devices enable continuous measurement while providing a high level of user comfort (22–25).
However, sweat can only be detected and analysed once it reaches the skin’s surface, posing a unique challenge for real-time and controlled collection. Current programmes typically rely on methods such as strenuous exercise, heating, pressure, and ionisation energy stimulation to elicit sweat, which are often unsuitable for diabetics. Efforts are underway to address these limitations and improve overall effectiveness.
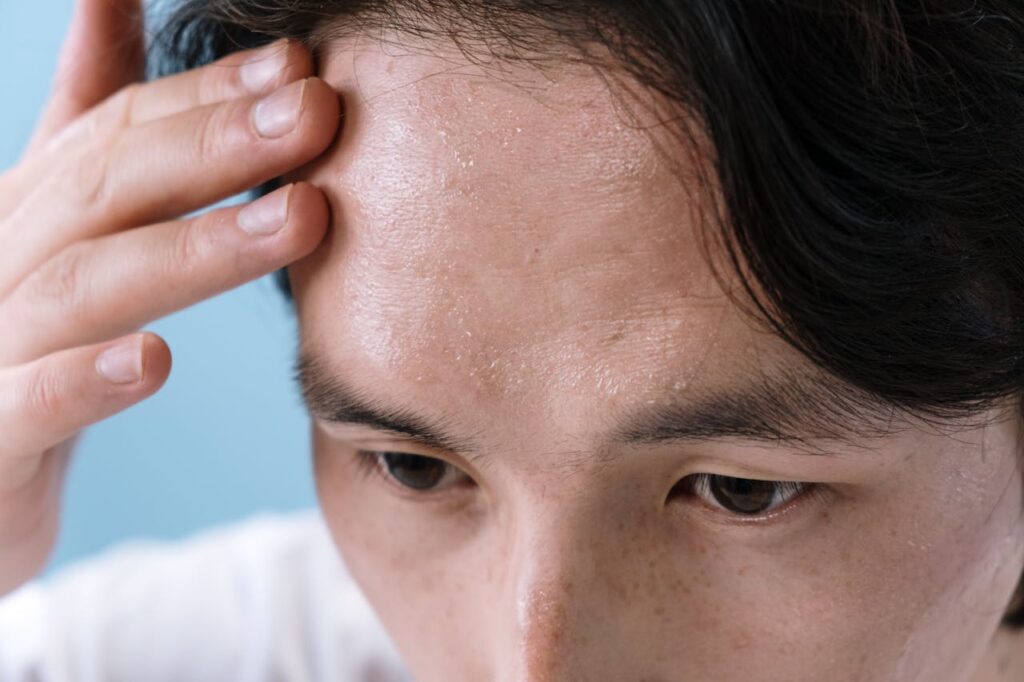
Finally, non-invasive glucose sensors based on interstitial fluid (ISF) have demonstrated considerable potential due to the elevated glucose concentrations in ISF and the strong correlation between ISF and plasma glucose levels (26–28). The direct diffusion capacity of glucose between the endothelium and blood vessels plays a crucial role in these sensors’ functionality. Various non-invasive ISF sampling methods, including sonophoresis, laser technology, and reverse iontophoresis (RI) (29–31), have been developed for glucose extraction.
RI is the most common technique for extracting ISF biomarkers through the skin. It uses a small electrical current to drive glucose molecules from the ISF to the skin’s surface, where they can be collected and analysed. This method offers high accuracy and is often more precise than other approaches. However, while higher currents can improve detection limits, they may irritate the skin, causing discomfort and reducing user satisfaction. Additionally, as there is a defined delay in material exchange between blood and ISF, measured results may lag, potentially not reflecting real-time glucose levels. Efforts are underway to improve this technique.
Glancing Forward: Innovations Shaping Non-Invasive Glucose Monitoring
When developing a glucose monitoring device, the accuracy and reliability of the output signal are critical, regardless of the technique used. The sensor’s performance is typically evaluated using key indicators such as sensitivity, detection range, detection limit, and response time.
Non-invasive blood glucose monitoring holds great promise for becoming more efficient, affordable, robust, and competitive, particularly with the rapid development of wearable technology. However, further advancements are necessary to ensure the accuracy and reliability of non-invasive continuous glucose monitoring systems.
Future research could improve the precision of non-invasive glucose measurement and its correlation with blood glucose levels for instance by integrating additional physical parameters (e.g., pH, temperature, humidity, frequency) and other glucose-related biomarkers. Achieving this will require the integration of multiple functional modules into wearable devices and interdisciplinary collaboration across fields such as materials science, engineering, medicine, molecular biology, electronics, informatics, and other relevant disciplines.
Bibliography:
- Khan MAB, Hashim MJ, King JK, Govender RD, Mustafa H, Al Kaabi J. Epidemiology of Type 2 Diabetes – Global Burden of Disease and Forecasted Trends. J Epidemiol Glob Health. 2020;10(1):107-111.
- Tinajero MG, Malik VS. An Update on the Epidemiology of Type 2 Diabetes: A Global Perspective. Endocrinol Metab Clin North Am. 2021;50(3):337-355.
- American Diabetes Association. Classification and Diagnosis of Diabetes. Diabetes Care. 2015; 38:S8–S16.
- Bailes BK. Diabetes mellitus and its chronic complications. AORN J. 2002;76(2):266-286.
- American Diabetes Association. Standards of medical care in diabetes—2014. Diabetes Care 2014, 37 (Suppl. 1), S14–S80.
- Tang L, Chang SJ, Chen CJ, Liu JT. Non-Invasive Blood Glucose Monitoring Technology: A Review. Sensors (Basel). 2020;20(23):6925.
- Johnston, L.; Wang, G.; Hu, K.; Qian, C.; Liu, G. Advances in Biosensors for Continuous Glucose Monitoring Towards Wearables. Front. Bioeng. Biotechnol. 2021, 9, No. 733810.
- Bent, B.; Cho, P. J.; Wittmann, A.; Thacker, C.; Muppidi, S.; Snyder, M.; Crowley, M. J.; Feinglos, M.; Dunn, J. P. Non-Invasive Wearables for Remote Monitoring of Hba1c and Glucose Variability: Proof of Concept. BMJ. Open Diab. Res. Ca. 2021, 9 (1), No. e002027.
- Liu, S.; Shen, Z.; Deng, L.; Liu, G. Smartphone Assisted Portable Biochip for Non-Invasive Simultaneous Monitoring of Glucose and Insulin Towards Precise Diagnosis of Prediabetes/Diabetes. Biosens. Bioelectron. 2022, 209, No. 114251
- Li Y, Chen Y. Review of Noninvasive Continuous Glucose Monitoring in Diabetics. ACS Sens. 2023;8(10):3659-3679.
- Malon, R.S.; Sadir, S.; Balakrishnan, M.; Corcoles, E.P. Saliva-based biosensors: Noninvasive monitoring tool for clinical diagnostics. BioMed Res. Int. 2014, 2014, 962903.
- Zhang, W.; Du, Y.; Wang, M. L. Noninvasive Glucose Monitoring Using Saliva Nano-Biosensor. Sensing and Bio-Sensing Research 2015, 4, 23−29.
- Bordbar MM, Hosseini MS, Sheini A, et al. Monitoring saliva compositions for non-invasive detection of diabetes using a colorimetric-based multiple sensor. Sci Rep. 2023;13(1):16174.
- Zhang Z, Wang S, Liu G, et al. Antifouling hydrogel film based on a sandwich array for salivary glucose monitoring. RSC Adv. 2021;11(44):27561-27569.
- Zhang Z, Dou Q, Wang S, et al. The development of an antifouling interpenetrating polymer network hydrogel film for salivary glucose monitoring. Nanoscale. 2020;12(44):22787-22797.
- Kownacka, A.E.; Vegelyte, D.; Joosse, M.; Anton, N.; Toebes, B.J.; Lauko, J.; Buzzacchera, I.; Lipinska, K.; Wilson, D.A.; Geelhoed-Duijvestijn, N.; et al. Clinical Evidence for Use of a Noninvasive Biosensor for Tear Glucose as an Alternative to Painful Finger-Prick for Diabetes Management Utilizing a Biopolymer Coating. Biomacromolecules 2018, 19, 4504–4511.
- Chu MX, Miyajima K, Takahashi D, et al. Soft contact lens biosensor for in situ monitoring of tear glucose as non-invasive blood sugar assessment. Talanta. 2011;83(3):960-965.
- Yao H, Shum AJ, Cowan M, Lähdesmäki I, Parviz BA. A contact lens with embedded sensor for monitoring tear glucose level. Biosens Bioelectron. 2011;26(7):3290-3296.
- Badugu R, Reece EA, Lakowicz JR. Glucose-sensitive silicone hydrogel contact lens toward tear glucose monitoring. J Biomed Opt. 2018;23(5):1-9.
- Park J, Kim J, Kim SY, Cheong WH, Jang J, Park YG, Na K, Kim YT, Heo JH, Lee CY, Lee JH, Bien F, Park JU. Soft, smart contact lenses with integrations of wireless circuits, glucose sensors, and displays. Sci Adv. 2018 Jan 24;4(1):eaap9841.
- Zafar H, Channa A, Jeoti V, Stojanović GM. Comprehensive Review on Wearable Sweat-Glucose Sensors for Continuous Glucose Monitoring. Sensors (Basel). 2022;22(2):638.
- Xu W, Lu L, He Y, Cheng L, Liu A. Long-Term Detection of Glycemic Glucose/Hypoglycemia by Microfluidic Sweat Monitoring Patch. Biosensors (Basel). 2024;14(6):294.
- Karim Z, Khan MJ, Hussain A, Ahmed F, Khan ZH. Multilayer patch functionalized microfibrillated cellulosic paper sensor for sweat glucose monitoring. Sci Rep. 2024;14(1):23434.
- Zhao H, Zhang L, Deng T, Li C. Microfluidic Sensing Textile for Continuous Monitoring of Sweat Glucose at Rest. ACS Appl Mater Interfaces. 2024;16(15):19605-19614.
- Chen Y, Sun Y, Li Y, et al. A wearable non-enzymatic sensor for continuous monitoring of glucose in human sweat. Talanta. 2024;278:126499.
- Bandodkar AJ, Jia W, Yardımcı C, Wang X, Ramirez J, Wang J. Tattoo-based noninvasive glucose monitoring: a proof-of-concept study. Anal Chem. 2015;87(1):394-398.
- Kim J, Sempionatto JR, Imani S, et al. Simultaneous Monitoring of Sweat and Interstitial Fluid Using a Single Wearable Biosensor Platform. Adv Sci (Weinh). 2018;5(10):1800880.
- Bantle JP, Thomas W. Glucose measurement in patients with diabetes mellitus with dermal interstitial fluid. J Lab Clin Med. 1997;130(4):436-441.
- Kost J, Mitragotri S, Gabbay RA, Pishko M, Langer R. Transdermal monitoring of glucose and other analytes using ultrasound. Nat Med. 2000;6(3):347-350.
- Pleitez M, von Lilienfeld-Toal H, Mäntele W. Infrared spectroscopic analysis of human interstitial fluid in vitro and in vivo using FT-IR spectroscopy and pulsed quantum cascade lasers (QCL): Establishing a new approach to non invasive glucose measurement. Spectrochim Acta A Mol Biomol Spectrosc. 2012;85(1):61-65.
- Pu Z, Zhang X, Yu H, et al. A thermal activated and differential self-calibrated flexible epidermal biomicrofluidic device for wearable accurate blood glucose monitoring. Sci Adv. 2021;7(5):eabd0199.